Recombinant Protein Purification Methods
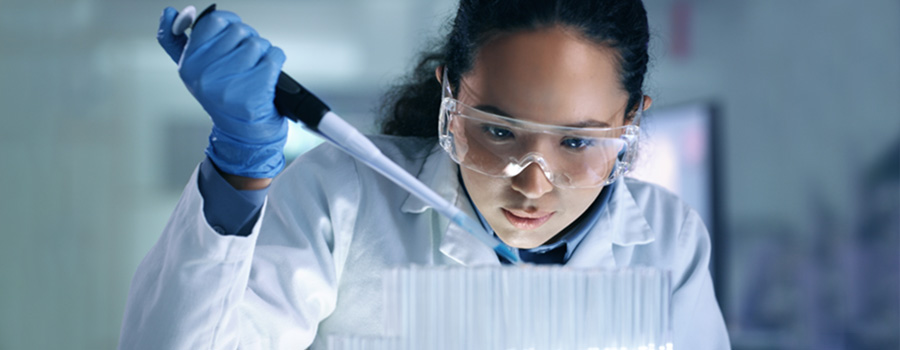
Recombinant protein purification methods are important in modern biotechnology to enable specific proteins for various applications, such as research, diagnostics and therapeutics. With advancements in genetic engineering, scientists can produce proteins of interest in heterologous host systems, such as bacteria, yeast or mammalian cells.
However, these expression systems often yield complex mixtures of cellular components alongside the target protein. As a result, efficient purification techniques are required to isolate the desired protein in a highly pure and functional form. This involves a multistep that requires cell lysis, clarification of lysates, selective capture using chromatography, and ensuring removal of impurities.
What is recombinant protein purification?
Recombinant protein purification is the process of isolating and purifying proteins that have been produced using recombinant DNA technology. It involves separating the target protein of interest from a complex mixture of other cellular components and impurities, such as host cell proteins, nucleic acids and culture media components.
The purification process typically employs techniques such as chromatography , precipitation, filtration and centrifugation, to selectively separate and purify the recombinant protein based on its physicochemical properties and interactions with specific ligands or matrices. The goal of recombinant protein purification is to obtain a highly pure, bioactive and homogeneous protein sample for further characterization, structural studies, therapeutic applications or other downstream processes.
Importance of Protein Purification in Biopharma Research
Protein purification is important in biopharmaceutical research and development. It is a fundamental process that allows scientists to isolate and obtain highly pure proteins from complex biological samples. The importance of protein purification in research and biotech applications can be explained as follows:
- Study of Protein Structure and Function
It is important to purify the proteins to study their structure and function. By obtaining pure proteins, researchers can characterize their properties, including their three-dimensional structure, enzymatic activity, binding affinity, and molecular interactions. This knowledge helps understand vital protein function, elucidating biological mechanisms and designing targeted therapies.
- Drug Discovery and Development
Protein purification is a key step in drug discovery and development. Many therapeutic drugs are proteins or protein-based molecules, such as antibodies, enzymes and cytokines. Purification allows scientists to obtain these proteins in their active and highly pure form, which helps in the accurate assessment of their therapeutic potential. Purified proteins are used for in vitro and in vivo studies to evaluate drug efficacy, selectivity and safety. This helps develop novel treatments for various diseases.
- Diagnostic Assays and Biomarker Discovery
Purified proteins can help develop diagnostic assays and identify biomarkers. Diagnostic tests often rely on specific protein interactions, such as antigen-antibody binding. By purifying the target proteins, researchers can ensure the accuracy and reliability of diagnostic assays, enabling early disease detection, monitoring of treatment response, and patient stratification. Additionally, purified proteins are crucial in biomarker discovery studies, where specific proteins indicate disease presence, progression or response to therapy.
- Protein Engineering and Biotechnology
Protein purification is a critical step in protein engineering and biotechnology applications. Purified proteins are modified or engineered to enhance their properties, such as stability, solubility or specificity. These engineered proteins find applications in various fields, including biocatalysis, industrial enzyme production, biosensors and bioremediation. Purification enables researchers to obtain highly pure protein samples for subsequent engineering, optimization and development of biotechnological products and processes.
- Structural Biology and Drug Design
Protein purification is vital for structural biology and drug design efforts. As a result, it is important to determine the three-dimensional structure of proteins for rational drug design, as it provides insights into ligand binding sites and protein-drug interactions. Purified proteins are used in techniques such as X-ray crystallography, cryo-electron microscopy and nuclear magnetic resonance (NMR) spectroscopy to solve protein structures. These structural insights help design and optimize small-molecule drugs and therapeutic antibodies.
Traditional affinity chromatography
Affinity chromatography is a highly effective method used for purifying recombinant proteins by exploiting their specific interactions with immobilized ligands. This technique utilizes the strong affinity between a desired protein and a particular ligand, enabling selective and efficient purification.
The ligand is immobilized onto a solid support material, such as a resin or column matrix. When a mixture containing the target protein is passed through the column, the protein of interest selectively binds to the immobilized ligand due to its specific recognition properties. Contaminants are then washed away, and the purified protein is eluted. This yields a highly pure sample for various research, diagnostics and biotechnology applications.
The step-by-step procedure for purifying recombinant proteins using traditional affinity chromatography typically involves the following key stages:
- Ligand Immobilization
The ligand, which possesses high affinity and specificity for the target protein, is covalently attached to a solid matrix. Agarose beads, Sepharose or magnetic beads are some commonly used matrices.
- Equilibration
The immobilized matrix is equilibrated with an appropriate buffer system to create optimal conditions for protein binding and subsequent purification. The buffer composition is selected based on the properties of the target protein and the ligand.
- Sample Loading
The mixture containing the crude protein extract or the sample of interest is applied to the column containing the immobilized ligand. The sample is allowed to interact with the ligand, enabling the target protein to selectively bind to the ligand while nonspecific proteins and contaminants pass through.
- Washing
After the sample loading, the column is washed with a buffer solution to remove any unbound impurities, nonspecific proteins and other contaminants. This step helps in achieving the high purity of the target protein.
- Elution
The bound target protein is then eluted from the column using a specific elution buffer. The elution buffer disrupts the protein-ligand interaction, allowing the target protein to be released from the column. Elution conditions, such as pH, salt concentration or the addition of competitive ligands, can be adjusted to optimize the purification yield and purity.
- Analysis and Further Purification
The eluted protein fractions are collected, and the purity and concentration of the purified protein are analyzed using various techniques, such as SDS-PAGE, Western blotting or spectrophotometry. If necessary, additional purification steps, such as size-exclusion chromatography or ion-exchange chromatography, can be employed to refine the protein sample further.
Advantages of Traditional Affinity Chromatography
Traditional affinity chromatography provides a robust and effective method for purifying recombinant proteins with high specificity, purity and versatility. As a result, it is a widely used technique in protein purification. Here are the advantages of traditional affinity chromatography.
High Specificity
Affinity chromatography stands out for its remarkable specificity in purifying target proteins. The technique utilizes ligands possessing a strong affinity for the desired protein, ensuring selective binding while minimizing interactions with nontarget proteins. This level of specificity proves particularly valuable when dealing with complex mixtures like crude cell lysates, where numerous proteins may coexist. By employing ligands that specifically recognize the target protein, affinity chromatography helps isolate the desired protein with a high degree of selectivity, facilitating downstream applications.
High Purity
One of the significant advantages of affinity chromatography is its ability to deliver highly purified protein samples. By using the specific interaction between the target protein and the immobilized ligand, nonspecific proteins and contaminants can be effectively washed away during the purification process. This results in a purified protein sample with minimal impurities that ensures the integrity of downstream analyses and experiments. The high purity obtained through affinity chromatography serves well in applications that require accurate characterization and functional studies of the purified protein.
Gentle Purification Conditions
Affinity chromatography is known for its gentle purification conditions, which help maintain the target protein’s activity and stability. The technique can be conducted under mild conditions, minimizing the risk of protein denaturation or loss of biological function. By preserving the native conformation and activity of the protein, affinity chromatography provides a favorable environment for downstream applications that rely on the functional integrity of the purified protein. This gentle purification approach is particularly beneficial for fragile or sensitive proteins that may be susceptible to harsh purification methods.
Size-exclusion chromatography (SEC)
Size-exclusion chromatography (also called gel filtration chromatography or gel permeation chromatography) is a commonly employed method for purifying and separating biomolecules based on their size. This technique relies on the principle of differential partitioning, utilizing a porous stationary phase.
As the sample mixture is applied to the column, smaller molecules penetrate the pores and travel a longer path, resulting in delayed elution, while larger molecules are excluded and elute earlier. This differential partitioning allows for the separation of biomolecules according to their size, with the larger molecules being eluted first and the smaller ones later. SEC is widely utilized in protein purification, characterization and the analysis of macromolecular complexes.
The procedure for purifying recombinant proteins using SEC typically involves the following steps:
- Column Selection
Choose an SEC column with an appropriate pore size that allows the separation of the target protein from other components in the sample. The pore size should be selected based on the target protein’s size range and the column’s molecular weight cutoff.
- Column Equilibration
Before sample loading, the SEC column is equilibrated with a buffer that matches the desired conditions for the protein of interest. The equilibration buffer typically contains suitable salts, pH and additives to maintain protein stability.
- Sample Loading
Prepare the sample by removing any particulates or aggregates through centrifugation or filtration. Load the sample onto the equilibrated SEC column. As the sample passes through the column, larger molecules elute faster due to their ability to bypass the pores, while smaller molecules, including the target protein, will take longer to traverse the column.
- Elution and Fraction Collection
As the sample elutes from the column, different components are separated based on their size. Fractions are collected using a fraction collector or manually by collecting distinct peaks corresponding to different proteins or biomolecules. The elution profile can be monitored by measuring absorbance at specific wavelengths or using other detection methods.
- Analysis and Characterization
Analyze the collected fractions using techniques such as SDS-PAGE, Western blotting or spectrophotometry to determine the presence and purity of the target protein. The fractions containing the purified protein are pooled and concentrated if necessary.
Advantages of Size Exclusion Chromatography
Compared to affinity chromatography, SEC offers unique benefits by utilizing size-based separation. This provides versatility in sample compatibility, ensures gentle purification conditions and efficiently removes aggregates and contaminants. These advantages make SEC a valuable tool for protein purification, especially when specific ligands are not available or when size differences are critical for separation.
Size-Based Separation
SEC offers a significant advantage in separating biomolecules based on their size. Unlike affinity chromatography, which relies on specific interactions, SEC relies solely on the molecular size of the biomolecules. This makes SEC applicable to a wide range of proteins, as it does not require specific ligands or affinity tags. By exploiting the differential partitioning of molecules through the porous stationary phase, SEC effectively separates proteins based on their size, allowing for the purification of proteins without the need for additional specific interactions.
Versatility in Sample Compatibility
SEC is a versatile technique that can accommodate various sample types. It can effectively handle crude samples, such as cell lysates or complex mixtures, without significant interference from contaminants or impurities. Moreover, SEC is compatible with proteins of different molecular weights, ranging from small peptides to large protein complexes. This versatility makes SEC suitable for purifying a wide range of biomolecules, enabling researchers to obtain pure samples from diverse sources and applications.
Gentle Purification Conditions
SEC operates under mild conditions, ensuring minimal denaturation or damage to the target protein. Unlike affinity chromatography, which may involve harsh elution conditions or affinity tag cleavage, SEC does not require aggressive chemical treatments or high temperatures. The gentle purification conditions of SEC help preserve the protein’s native conformation, activity and stability. This is particularly advantageous when working with fragile or sensitive proteins that are susceptible to denaturation or loss of functionality. By maintaining the protein’s integrity, SEC allows for downstream applications that require functional and structurally intact proteins.
Ion-exchange chromatography (IEC)
Ion-exchange chromatography is an extensively employed method for purifying and separating proteins based on their net charge. This technique leverages the reversible interaction between charged proteins and functional groups on a stationary phase.
The stationary phase typically contains either positively or negatively charged groups, while the proteins in the sample possess the opposite charge. As the sample is applied to the column, proteins with a charge opposite to that of the stationary phase selectively bind, while proteins with similar charges pass through.
The step-by-step procedure for purifying recombinant proteins using IEC typically involves the following key stages:
- Selection of Ion-Exchange Resin
Choose an appropriate ion-exchange resin based on the properties of the target protein and the desired purification conditions. Anion-exchange chromatography (AEC) is used when the target protein carries a net negative charge, while cation-exchange chromatography (CEC) is employed for proteins with a net positive charge.
- Equilibration
The ion-exchange column is equilibrated with an equilibration buffer that matches the desired pH, ionic strength and buffering capacity. The equilibration buffer establishes the appropriate conditions for protein binding to the ion-exchange resin.
- Sample Loading
Prepare the protein sample by adjusting its pH and ionic strength to facilitate binding to the ion-exchange resin. Load the sample onto the equilibrated column, and the charged proteins interact with the oppositely charged functional groups on the resin. The degree of protein binding depends on the net charge, pH and ionic strength of the protein and the ion-exchange resin.
- Washing
After sample loading, the column is washed with a wash buffer to remove nonspecifically bound proteins and impurities. The wash buffer typically has a higher ionic strength than the equilibration buffer, allowing for the selective removal of weakly bound proteins while retaining the target protein on the column.
- Elution
The bound proteins are eluted from the column by changing the buffer conditions. This can be achieved by altering the pH and ionic strength or by using gradient elution. Elution disrupts the protein-ion-exchange resin interaction, allowing the target protein to be released. The eluted fractions containing the purified protein are collected.
- Analysis and further purification
Analyze the collected fractions to assess the purity and concentration of the target protein using techniques like SDS-PAGE, Western blotting or spectrophotometry. If necessary, additional purification steps, such as size-exclusion chromatography or affinity chromatography, can be employed for further refinement of the protein sample.
Here are the specific uses of IEC in protein purification:
- Protein Separation and Purification
IEC is commonly employed to separate and purify proteins from complex mixtures. It is particularly useful when the target protein of interest has a specific charge property that can be exploited for selective binding to the ion-exchange resin. By utilizing the reversible binding of charged proteins to the resin, IEC allows for the isolation and purification of the target protein from impurities.
- Fractionation of Protein Mixtures
IEC can be used to fractionate protein mixtures based on their charge properties. Different proteins have varying degrees of charge and will interact differently with the ion-exchange resin. By adjusting the ionic strength or pH of the elution buffer, proteins can be eluted from the column at different stages, leading to their separation into distinct fractions. This fractionation step can be valuable for analyzing protein composition or studying protein-protein interactions.
- Polishing Step in Protein Purification
IEC is often employed as a polishing step in protein purification workflows. After initial purification steps, such as affinity chromatography or size-exclusion chromatography, residual impurities may still be present in the protein sample. IEC can effectively remove these contaminants by exploiting the differences in their charge properties. The target protein can be retained on the column while impurities are washed away, resulting in a highly purified protein sample.
Advantages of Ion-Exchange Chromatography
IEC offers several advantages in protein purification , including gentle purification conditions, high resolution and compatibility with downstream applications. These advantages make IEC a valuable tool for researchers and industrial applications seeking to obtain pure and functional proteins from complex mixtures. Here are the key advantages of IEC:
Gentle Purification Conditions
IEC offers the advantage of gentle purification conditions, which are typically nondenaturing and nondestructive to proteins. This is particularly beneficial for delicate or sensitive proteins that may be prone to denaturation or degradation under harsh conditions. By maintaining the native conformation and activity of the proteins, IEC ensures the production of biologically active and functional proteins, making it a preferred method for purifying proteins without compromising their structural integrity.
High Resolution
IEC can achieve high resolution in protein separation due to the availability of a diverse range of ion-exchange resins with varying charge densities and selectivities. This versatility allows for fine-tuning of the separation process, resulting in excellent resolution and effective separation of closely related proteins or protein isoforms. The high-resolution capability of IEC is particularly valuable in research and analytical applications where precise protein characterization and analysis are crucial for obtaining accurate results.
Compatibility With Downstream Applications
Proteins purified using IEC are generally compatible with various downstream applications. The gentle purification conditions employed by IEC help maintain the biological activity and integrity of the proteins, ensuring their functionality in subsequent experiments and applications. This compatibility extends to enzymatic assays, structural studies, protein-protein interaction studies and other functional assays where the purified protein’s native state and activity are vital. The versatility of IEC enables researchers to obtain high-quality protein samples that can be effectively utilized in various downstream applications.
Comparative analysis of methods
Here’s a comparison table summarizing the key features, advantages and limitations of three common protein analysis methods. It also includes an evaluation of factors influencing method selection.
Method | Key Features | Advantages | Limitations | Factors Influencing Method Selection |
---|---|---|---|---|
Affinity Chromatography | Uses specific ligands for target protein purification |
|
|
|
Size-Exclusion Chromatography | Separates proteins based on size and molecular weight |
|
|
|
Ion-Exchange Chromatography | Separates proteins based on charge differences |
|
|
|
Conclusion
In conclusion, it is important to select an appropriate purification method for successful downstream processing in biopharmaceutical research. It is also essential to consider specific research needs, such as target molecule characteristics, impurity profile, scalability and cost-effectiveness, when choosing a purification method. Furthermore, future advancements and emerging techniques in recombinant protein purification hold great potential for improving efficiency and productivity.
These advancements include the development of novel chromatographic resins, affinity tags, membrane-based separations and continuous purification processes. By leveraging these emerging techniques and staying abreast of advancements in the field, researchers can enhance the purification of recombinant proteins, streamline downstream processes and pave the way for developing innovative biopharmaceutical products that address unmet medical needs.