Cell and Gene Therapy Therapeutic Mechanisms and Strategies
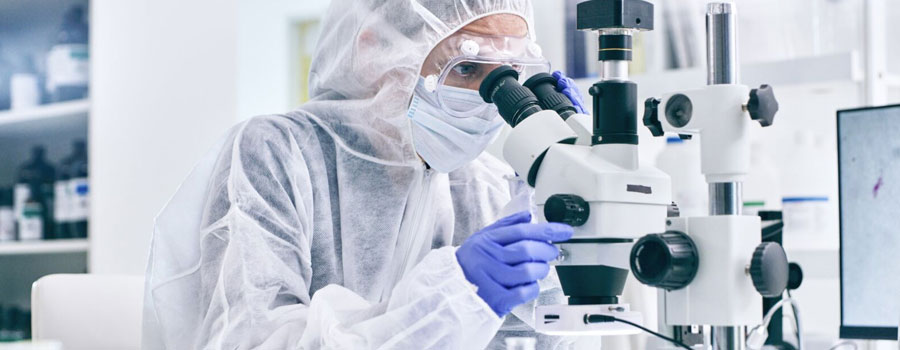
Gene and cell therapy have emerged as groundbreaking methods for treating diverse diseases through genetic engineering and/or cellular reprogramming. Various gene and cell therapy mechanisms and strategies are utilized in these innovative approaches.
By examining gene editing techniques and the manipulation of stem cells, it’s possible to gain a comprehensive understanding of the intricate workings of these therapies. Besides, gene and cell therapy has the potential to transform healthcare by offering targeted interventions for a wide range of medical conditions.
The intersection of gene and cell therapy
Gene therapy and cell therapy represent two distinct yet interconnected fields in the world of biomedical research. Gene therapy involves modifying or adding genes to a patient’s cells in vivo to correct genetic abnormalities.
On the other hand, cell therapy has traditionally focused on using patient or donor cells to treat blood cancers or replace the entire hematopoietic system using hematopoietic stem cells or lymphocytes (e.g., bone marrow transplant) or has used mesenchymal or epithelial stem cells to repair a variety of tissues, such as those of skeletal, muscle and circulatory systems.
The convergence of gene therapy and cell therapy has evolved over the past two decades with gene therapy principles integrated with cell-based approaches. This fusion is achieved through the ex vivo manipulation of patient-derived or donor cells, resulting in improved precision for cancer targeting, as represented by chimeric antigen receptor T-cell (CAR-T) therapy. Additionally, this synergy enables the introduction of functional genes that may be challenging to deliver in vivo via conventional gene therapy methods.
Current research in this area aims to refine delivery systems, such as viral vectors or nanoparticles, to transport therapeutic genes into target cells efficiently. Additionally, scientists are exploring the potential of gene editing technologies, like CRISPR-Cas9 , to correct a patient’s gene sequence instead of adding a whole new gene.
Gene therapy mechanisms
Gene therapy mechanisms aim at delivering therapeutic genes into target cells to correct genetic defects. The delivery of genes can be achieved through different approaches, including viral vectors and nonviral methods such as lipid nanoparticles.
Once inside the target cells, the new genes can integrate into the genome or remain separate depending on the desired outcome. However, gene therapy faces challenges, such as immune responses against vectors, limited efficiency of gene transfer, and off-target effects. It is essential to overcome the obstacles to realize the full potential of gene therapy as a transformative medical intervention.
The Different Approaches to Gene Delivery
Gene delivery involves several approaches, each with its own advantages, limitations and considerations. These variations allow therapeutic developers to choose the most suitable method based on factors such as target cell type, desired gene expression duration, delivery efficiency, safety profile and regulatory considerations. Depending on the application, such as systemic (IV) delivery or injection, viral vectors like adeno-associated viruses (AAVs), adenoviruses (AVs) and parvovirus are commonly used. Nonviral vectors are currently employed clinically for specific ex vivo cell treatments or highly targeted tissue injections.
Viral Vectors
Viral vectors are modified viruses used to deliver therapeutic genes into cells. They include AVs (efficient for diverse cells), HIV-derived lentiviruses (effective for dividing and nondividing cells) and AAVs, with long-term gene expression and favorable safety profile. While viral vectors offer high transduction efficiency, they have limitations, like immunogenicity, size constraints, mutagenesis risks and manufacturing difficulties.
Nonviral Vectors
Nonviral vectors are synthetic delivery systems without viral components and are considered safer and easier to produce at scale. Common approaches include lipid-based systems like liposomes and lipid nanoparticles, which efficiently deliver genes but may have low transfection efficiency in non-dividing cells.
Polymer-based systems like PEI and PLGA form complexes with DNA or RNA for controlled vector properties and targeting specific cells or tissues. However, their transfection efficiency may be lower than viral vectors. Nonviral vectors offer safety advantages but have so far faced challenges in achieving high transfection rates.
Techniques for Integrating New Genes Into Target Cells
Gene integration refers to the process by which a foreign gene is permanently incorporated into the genetic material (genome) of a host organism. This integration enables the stable expression of the gene. It is an important step in gene therapy and genetic engineering, and various techniques are employed for this purpose.
Viral-based methods, such as retroviral and lentiviral vectors, efficiently deliver genes into target cells and integrate them into the host genome. Retroviral vectors transfer genes into dividing cells, offering stable and long-term gene expression. Additionally, AAV and AV vectors have shown efficacy in targeting a wide range of cell types, with the advantage of remaining stable in the bloodstream. The serotype of AAV confers specificity and enables it to infect both dividing and nondividing cells.
However, AAV’s performance is compromised in immune cells, leading to the development of retroviral and lentiviral vectors that can overcome this limitation. Lentivirus, being a generation beyond retroviruses, has become a preferred choice for modern gene delivery due to its improved characteristics. On the other hand, nonviral methods provide an alternative approach, where plasmid DNA delivery uses carriers like liposomes or nanoparticles to introduce therapeutic genes into the nucleus.
Another powerful technique being developed for gene integration is the use of genome editing tools like CRISPR-Cas9 for precise modification of native DNA in the target cell’s genome. CRISPR-Cas9 allows targeted and controlled integration of new genes, cuts or additions into specific genomic loci. Certain factors to consider when choosing a gene integration technique include the target cell type, desired stability and duration of gene expression, integration efficiency and potential off-target effects.
Challenges in Gene Therapy
Gene therapy faces several challenges that need to be addressed for its widespread application. Immune responses, off-target effects, limited gene transfer efficiency, and regulatory considerations pose significant hurdles. Each challenge requires specific research efforts and innovative strategies to overcome them.
Immune Responses
One of the challenges in gene therapy is the immune response triggered by the therapeutic vector. Viral vectors, commonly used for gene delivery, can be recognized as foreign by the immune system, leading to defensive immune responses. This immune response can cause inflammation, destruction of viral particles and elimination of transduced cells, reducing the efficacy of gene therapy. Some strategies to mitigate immune responses include modifying viral vectors to make them less immunogenic or developing immune modulation techniques to suppress unwanted immune reactions. Additionally, alternative nonviral delivery systems are being explored to minimize immune responses.
Off-Target Effects
Off-target effects refer to unintended genetic modifications occurring at sites other than the intended target during gene therapy. This is particularly relevant when using gene editing tools like CRISPR-Cas9. It is important to ensure precise targeting and minimize off-target effects for the safety and accuracy of gene therapy. Ongoing research focuses on refining gene editing techniques, improving guide RNA design and developing novel editing tools with enhanced specificity. The goal is to achieve more precise gene editing and reduce the risk of unintended genetic alterations.
Limited Gene Transfer Efficiency
The efficient delivery of therapeutic genes to target cells is essential for the success of gene therapy. While viral vectors offer high transduction rates, they have limitations, such as restricted cargo capacity, and concerns about their immunogenicity. Different serotypes, like AAV8 or AAV9, exhibit tissue specificity, which can provide opportunities for enhanced targeting. Nonviral delivery systems, such as nanoparticle-based approaches and advanced lipid formulations, are being investigated to improve gene transfer efficiency. These nonviral vectors aim to enhance the targeted delivery of genes and overcome the limitations of viral vectors. Additionally, advancements in viral vector engineering seek to improve their specificity, capacity and transduction efficiency.
Cell therapy mechanisms
Cell therapy mechanisms involve the utilization of various types of cells for therapeutic purposes. The primary goal is to harness the potential of these cells to repair damaged tissues, enhance immune responses or administer therapeutic molecules. Stem and immune cells are commonly used in cell therapy, each with unique properties and functions. Isolation, expansion and manipulation techniques are employed to obtain the desired cell populations.
However, implementing cell therapy faces challenges such as safety, efficacy and scalability. Issues such as immune rejection, limited cell survival, potential tumorigenicity and complex cell and gene therapy manufacturing processes must be overcome to ensure cell-based therapies’ successful and widespread adoption.
Types of Immune Cells Used in Cell Therapy
Each type of cell used in cell therapy has its own characteristics, advantages and limitations. The choice of cell type depends on factors such as the specific therapeutic application, the desired differentiation potential, immunogenicity, scalability and safety considerations for clinical use. Here are the types of cells used in cell therapy.
Induced Pluripotent Stem Cells (iPSCs)
iPSCs are generated by reprogramming adult somatic cells, such as skin cells, into a pluripotent state. They share similar characteristics with ESCs, including the ability to differentiate into various cell types. iPSCs overcome the ethical concerns associated with ESCs since they are not derived from embryos. However, there are challenges regarding the efficiency of reprogramming and the potential for genetic abnormalities during the reprogramming process.
Mesenchymal Stem Cells (MSCs)
MSCs are adult stem cells that can be isolated from various tissues, including bone marrow, adipose tissue and umbilical cord blood. They can differentiate into multiple cell lineages and possess immunomodulatory and regenerative properties. MSCs are considered beneficial for cell therapy due to their relative ease of isolation and expansion, low immunogenicity and potential for tissue repair. However, their differentiation capacity may be limited compared to pluripotent stem cells.
Hematopoietic Stem Cells (HSCs)
HSCs are primarily found in the bone marrow and generate different blood cell types. They have been widely used in hematopoietic stem cell transplantation (HSCT) to treat various blood disorders and cancers. HSCs can be harvested from the bone marrow or mobilized into the peripheral blood for collection. HSCT using HSCs from a compatible donor can replace the patient’s diseased or damaged hematopoietic system. However, finding a suitable donor match can be challenging, and there are risks of graft-versus-host disease and transplant rejection.
Mature Differentiated Immune Cells
Therapies such as CAR-T or cellular vaccines utilize immune cells that have been modified to target cancer or activate the immune system. For CAR-T, mature immune cells are harvested from the patient (autologous) or donor (allogeneic) by apheresis and are genetically engineered to express chimeric antigen receptors (CARs).
These receptors enable T-cells to recognize and attack specific cancer cells when infused back into the patient. Natural killer (NK) cells, Natural killer T (NKT) cells and tumor-infiltrating lymphocytes (TIL) can also be engineered for similar purposes. CAR-T therapies have shown remarkable success against certain blood cancers, offering targeted and potent treatments. However, challenges include managing cytokine release syndrome, neurotoxicity and expanding this approach to solid tumors while maintaining safety and efficacy.
Methods for Isolating, Expanding and Manipulating Target Cells
Maintaining cell viability, functionality and safety is of utmost importance during the isolation, expansion and manipulation of target cells. Factors such as aseptic techniques, appropriate culture conditions, monitoring cell quality and ensuring the absence of contaminants are critical considerations throughout the process.
Tissue, Plasma and Bone Marrow Harvest
Cellular starting material can be harvested from solid tissue, bone marrow or plasma. Tissue biopsy involves the extraction of a small sample of tissue from a patient. The source material for nonhematopoietic therapies is often a small subset of cells contained within various tissues, including adipose, muscle and epithelium. Collection of these tissue samples can be done with techniques such as needle, punch and surgical biopsies.
The hematopoietic system originates from bone marrow, and therefore bone marrow is used for complete immune system/hematological system replacement. Bone marrow is most commonly harvested from donors using a needle biopsy from the crest of the hip bone. For cellular immunotherapies, including CAR therapies, plasma (rich in mature immune cells) is harvested by apheresis.
Cell Culture
Cell culture involves the in vitro growth and expansion of isolated cells in a controlled environment. It provides a means to propagate and amplify the desired target cells for cell therapy. When called for, cells are cultured in specialized media containing growth factors, nutrients and supplements. This method allows therapeutic manufacturers to obtain a larger number of cells and maintain their viability and functionality.
Cell Sorting
Cell sorting techniques, such as flow cytometry and magnetic-activated cell sorting (MACS), can be used to isolate specific cell populations from a heterogeneous mixture. These methods rely on cell surface markers and specific labeling techniques to selectively separate target cells. Cell sorting allows for precise isolation and enrichment of the desired cell types, which is particularly important when working with rare or specialized cell populations.
Genetic Modification
Genetic modification techniques are employed to introduce specific genetic material into target cells for various purposes. Many methods are in development, including using viral or nonviral vectors to deliver therapeutic genes, editing the cells’ genome with techniques like CRISPR-Cas9, or altering gene expression through RNA interference. Genetic modification enables the manipulation of target cells to enhance their therapeutic potential, such as enhancing their homing ability and immune response.
Challenges in Cell Therapy
Despite being an effective therapeutic technique, cell therapy often faces several challenges that can be addressed with ongoing research and advancements. Collaboration between researchers, clinicians, regulatory bodies and industry stakeholders is crucial to overcome these challenges and harness the full potential of cell therapy. Here are the challenges in cell therapy.
Cell Sourcing
One of the primary challenges in cell therapy is obtaining a sufficient number of cells with the desired characteristics. While traditional cell therapy has often sourced cells from donors, resulting in classic availability issues, gene-modified cell therapy has shifted toward predominantly utilizing autologous cells.
However, a unique difficulty arises with gene-modified cell therapy where the patient’s illness leads to a compromised immune system, eliminating the concern of immune rejection but posing other challenges. The availability of suitable cell sources can still be limited, especially when working with specialized cell types, and this applies to both traditional and gene-modified approaches. Additionally, it is still important to identify and develop scalable cell sources to meet the increasing demand for various cell therapies.
Cell Characterization and Quality Control
The identity, purity and quality of cells used in therapy are paramount for ensuring safety and efficacy. It is important to use accurate characterization techniques to identify and quantify the desired cell populations and verify their functional properties. However, challenges emerge in establishing standardized protocols for characterizing cell products, which encompass defining suitable markers, evaluating cell viability and confirming the absence of contaminants. As a result, it is essential to implement robust quality control measures to minimize batch-to-batch variations and guarantee reproducibility in cell therapy.
Immune Rejection
In allogeneic cell therapy, the recipient’s immune response poses a significant challenge, as it can result in the rejection of transplanted cells. While immune rejection is more classical for bone marrow transplantation (BMT), it’s important to note that many modern cell therapies are designed to address this concern.
Newer cell therapies strive to be autologous, as they utilize a patient’s own cells or, in cases where donor cells are used, they are specifically engineered to prevent rejection by targeting and eliminating the human leukocyte antigens (HLAs) that trigger immune reactions. The antigens play a pivotal role in immune recognition, and any mismatch between the HLA types of the donor and recipient can trigger adverse immune responses.
To proactively tackle this challenge, various strategies are employed, such as rigorous HLA matching between donor and recipient, administration of immunosuppressive drugs to dampen immune reactions and genetic modification of cells to enhance immune tolerance. Despite these efforts, overcoming immune rejection remains a major hurdle in achieving long-term engraftment and favorable therapeutic outcomes in allogeneic cell therapy.
Conclusion
In conclusion, the development of gene and cell therapies is significant in advancing medical treatments. Gene therapy mechanisms encompass different approaches to gene delivery, techniques for integrating new genes into target cells and challenges in the field. Similarly, cell therapy mechanisms cover the types of cells utilized, methods for isolating, expanding and manipulating target cells, and the challenges faced in cell therapy. By understanding these therapeutic mechanisms and strategies, we can foster advancements in precision medicine and potentially revolutionize the treatment landscape for various genetic and acquired diseases. For more information on solving complex gene therapy workflows, visit Avantor.